The microstrip antenna is a convenient, planar, easy-to-fabricate antenna with some attractive attributes and features, as well as some distinct limitations.
- U Slot Patch Antenna Calculator Download
- 2 Meter Slot Antenna
- Square Patch Antenna Calculator
- Patch Antenna Design Calculator
The passive antenna is a critical part of any wireless communications link, for both transmit and receive sides. Depending on the application, the same antenna may be used for both roles, or radically different ones may be employed. This FAQ looks at antennas in brief and at a specific antenna embodiment: the microstrip (patch) antenna.
- A double U slot microstrip rectangular patch antenna with truncated corners is demonstrated in this paper. The antenna has been designed with FR4 substrate material whose dielectric constant is 4.
- Equivalent circuit of slot-loaded rectangular patch. The proposed microstrip patch antenna after inserting the microstrip line feed is shown in Figure 6. Hence, the total input impedance of the microstrip line feed slot-loaded rectangular patch can be calculated as ZM ZN ZP Z Ll3 Ll2 Cl1 Zl ZTotal s Figure 6. Equivalent circuit of.
Q: What are the functions and objectives of any antenna?
So design an microstrip patch antenna with small/compact size, light weight and good band width is an important issue. One of the techniques is design an antenna with Slot insertion in the ground plane. For this u slot of proper length is cut from ground plane at proper location. The size and position of the slot is very. Need a patch antenna design with u slot Once i tried to etch a U-slot on a well matched bottom-fed antenna, the S11 result turned to be extremely bad. Are there any guidelines for U-slot (or other shape of the slot) technique, which is used to enlarge the bandwidth of the patch antenna? Design of U-Slot Patch Antenna and its Geometry The U-slot patch antenna produces a wideband beam. The U-slot microstrip patch antenna is designed at 2.0 GHz on Arlon diclad 880 substrate (Ԑ r1 =2.2, h 1 =1.6 mm), using expressions available in the standard literature 1-6, 13, 14. The designed dimensions of U-slot patch antenna are given in.
A. An antenna is an energy transducer (Figure 1). A transmitting antenna takes electrical and electronic power (and energy) originating at an amplifier and which is in a confined space such as a wire or a waveguide and disperses (radiates) it as electromagnetic (EM) power into free space. A receiving antenna performs the complementary operation, capturing this EM energy in free space and confining it to a well-defined path (again, a wire or waveguide, as examples), then sending it to an RF front end.
Q: What are some of the key parameters which are used to characterize antennas?
A: As with any electronic component, whether active or passive, there are some top-tier factors which define performance. For antennas, these are:
- Center frequency
- Radiation pattern
- Radiation pattern bandwidth
- Sidelobe level
- Directivity
- Gain
- Efficiency
- Effective area
- Aperture efficiency
- Polarization
- Input impedance
The references explain these in more detail; some are 'intuitive,' while others require a deeper understanding of EM physics. One or more of these will be more dominant factors in design and selection than the others. Still, there is no formal ranking of their importance, as the relative ranking is a function of the application and its priorities.
Q: How does an antenna differ from many other passive components?
A: Antennas have tradeoffs (also called 'compromises') as do all components, but they often not so much related to compromise but instead focused on optimizing the antenna's match to the application. There is no such thing as an 'ideal' antenna because every application has its own priorities. For example, some applications need high directivity while others need the omnidirectional capability, Or some want wider bandwidth, and others need only narrower bandwidth. These are not tradeoffs or compromises: they are deliberate choices made to better-fit the antenna to the application needs. With antennas, there are many degrees of freedom to consider; some of these are tightly linked to each other, while others are more loosely linked.
Q: What does a typical antenna look like?
A: That question cannot be answered as there is no such thing as a 'typical' antenna. The classic whip or discrete wire antenna of decades ago, and often seen in movies, is just one of many physical antenna types (Figures 2 through 4).
Q: What does the antenna genealogy 'family tree' look like?
A: That's a simple question with a complicated answer, as it depends on the perspective of the person drawing that genealogy tree; Figure 5 is one example. Some classifications are based on the underlying EM physics principles. In contrast, others are derived from the actual physical realization, which is somewhat related to principles but not strictly defined by them.
Among the basic antenna groups, the earliest antenna belongs to what is called the 'wire' style (although often made of tubing or pipes in reality) such as the long wire, whip, and dipole antennas. Using very different embodiments of the same EM physics principles, there are small resonant antennas used in smartphones. There are also reflective antennas which use a parabolic dish or plane reflector behind the electrically connected main element, horn antennas, and microstrip antennas, to cite just a few basic types.
Q: Is that all?
A: Not at all. Antennas come in countless variations and permutations on their basic styles, and in many cases, are a blend of several types. For example, a parabola dish antenna may have a folded dipole or horn at its focal point. The many antenna physical realizations in use are clear proof of the art and ingenuity of designers as they work to meet specific goals and priorities. (If you need further evidence, take a look at some issues of QST, the flagship publication of the ARRL – the American Radio Relay League).

Q: How are antennas designed?
A: Decades ago, an antenna design started with an idea for the configuration and some basic analysis based on Maxwell's equations for the sizing of antenna elements, followed by building and testing. In recent years, the design mode has shifted radically to the designer establishing a configuration and then modeling it in detail using an 'EM field solver' application. These advanced applications take into account the real-world factors such as fringing and parasitics, which affect antenna dimensions, configuration, and performance, and which were nearly impossible to assess in advance using 'rules of thumb' and experience alone.
However, these advanced analysis and modeling applications cannot suggest radically new configurations; in most cases, that is done by leveraging and expanding on existing approaches or the intuition or experience of the designer. These hunches can then be verified and fine-tuned by the EM field-solver software and extended to 'what if?' modes and sensitivity analysis.
Q: What is a microstrip antenna?
A: A microstrip antenna, also called a patch antenna or printed antenna, is an antenna which is primarily a two-dimensional flat structure (Figure 6. In its simplest form, it uses a conducting 'patch' one-half wavelength long, so that the metal surface acts as a resonator similarly to the half-wave dipole antennas.
Q: Is that it, or is there more to the story?
A: Of course there is more! It is often built by simply mounting or fabricating a suitably dimensioned metal sheet or surface on an insulating dielectric substrate, such as a printed circuit board. The opposite side of the PC board substrate is also cladded and so forms a ground plane, or another conductive surface is added ion the other side of the dielectric. As a result, it is easy to design and inexpensive to manufacture. They can be built as standalone, discrete devices as a 'sandwich' of conductive material, dielectric, and conductive material as the ground plane.
Microstrip antennas are useful about 500 MHz to as high as 100 GHz; at the lower frequencies, they can be fairly large due to their half-wavelength dimension. As with many simple antennas, the basic EM theory and Maxwell's equations needed to model them start simple, but fringing, physical reality, tolerances, and parasitics play a large role in their actual performance.
Part 2 of this FAQ will explore the microstrip antenna in more detail, including its primary attributes, advantages and disadvantages, and variations.
EE World Related Content
Take a class in SDR and antenna design
The difference between active and passive antenna systems
Hidden pitfalls of IoT antenna design
New Antenna Concept Developed for Cars
What are RF waveguides? Part 2: implementation and components
What are RF waveguides? Part 1: context and principles
5 things you need to know about 5G filters
Passive microwave components, Part 2: couplers and splitters
Passive microwave components, Part 1: isolators and circulators
How do RFID tags and reader antennas work?
References and Resources
Basics and tutorials
- Maxtena, 'Microstrip Patch Antennas'
- IOP Conference Series: Materials Science and Engineering, 'Design, simulation and analysis: a microstrip antenna using PU-EFB substrate'
- Pasternack, 'Microstrip Patch Antenna Calculator'
- EU Radar Tutorial, 'Patch Antenna or Microstrip Antenna'
- Ranga Rodrigo, Arab Academy for Science, Technology, and Maritime Transport, 'Fundamental Parameters of Antennas'
- Pongsak University, Thailand, 'Chapter 2 : Fundamental parameters of antennas
- Tutorials Point, 'Antenna Theory – Micro Strip'
- Universal Engineering College, University of Calicut, 'Radiation and Propagation: Fundamentals of Antenna'
- ResearchGate, 'An Overview Of Microstrip Antenna'
- ResearchGate, 'Design of rectangular microstrip patch antenna'
- ResearchGate, 'Methods to Design Microstrip Antennas for Modern Applications'
- Science Direct, 'Microstrip Antenna'
- Science Direct, 'Stripline'
- Antenna-Theory.com, 'Microstrip (Patch) Antennas'
- Antenna-Theory.com,'PIFA – The Planar Inverted-F Antenna'
Advanced microstrip antenna implementations
- Elsevier, Science Direct, 'A Novel Compact Dual Frequency Microstrip Antenna'
- Elsevier, Journal of Applied Research and Technology, 'Design of triple-layer double U-slot patch antenna for wireless applications'
- ResearchGate, 'Methods to Design Microstrip Antennas for Modern Applications'
You may also like:
Services on Demand
- Cited by SciELO
Related links
- Similars in SciELO
On-line version ISSN 2179-1074
http://dx.doi.org/10.1590/2179-10742017v16i3867
Articles
Dual U-Slot Loaded Patch Antenna with a Modified L-Probe Feeding
1Department of Electronics and Communication Engineering, Raffles University, Neemrana, Rajasthan, INDIA-301705, Email: srakeshnath@gmail.com
2Department of Physics, Galgotias University, Greater Noida, Uttar Pradesh, INDIA-201308, Email: prabhakarsingh3@gmail.com
3School of Computational and Integrative Sciences, Jawaharlal Nehru University, New Delhi, INDIA-110067, Email: bkkanaujia@yahoo.co.in
In this paper, a modified L-strip fed patch antenna is theoretically analyzed for wideband applications. Dual U-shaped slots are incorporated in the radiating patch and a maximum bandwidth of 16.93% (2.65 GHz to 3.14 GHz) is achieved. Further, when two parasitic elements are used, antenna bandwidth improves up to 25.89% (2.90 GHz to 3.77 GHz). The maximum gain of dual U-slotted patch and with parasitic elements is 8.23 dBi and 8.46 dBi respectively. Antenna parameters are calculated by CST Microwave studio and equivalent circuit model theory is presented. The proposed antenna is fabricated and the measured results compare well with the theoretical as well as simulated results.
Index Terms Dual U-slot patch antenna; modified L-probe; multilayer patch antenna; gap coupled patch antenna; parasitic elements
I. INTRODUCTION
With the rapid development and attractive solution for various wireless communication systems and demands of their applications, compact and wideband antenna designs have been given immense priority [1], [2]. In this process, various methods were used to improve the bandwidth such as by loading the slots of different size and shape, etching notches and introducing discontinuities in the radiating patch as well as in the ground plane [3], [4]. There are several structures reported to improve the antenna characteristics such as E-shaped [5], [6], C-shaped, U-slot loaded and modified L-strip [7-9]. Different feeding methods also increase the antenna bandwidth such as proximity feed patch, asymmetric CPW fed patch antennas [10], [11]. Substrate with low dielectric constants, multilayer structures and use of air gaps between the dielectric layers increases the impedance bandwidth and gain of the microstrip antennas [12-14]. Besides the fed patch, some parasitic inverted-L wire improves the radiation performance of patch antennas [15], [16]. Apart from that some other types of parasitic element design such as slot type, shorted strip type etc [17], [18] can improve the antenna bandwidth and gain.
The present paper reports a radiating structure to study the antenna bandwidth, gain, efficiency and the radiation pattern. Dual U-slot is incorporated in the radiating patch such that one U-slot is lying within another U-slot forming dual U-slot loaded patch antenna (DUSPA). Further, to increase the bandwidth, two parasitic elements are used above this DUSPA. Both designs are fed by modified L-probe. All the calculations are made by using CST Microwave studio. Also, a theoretical analysis for the proposed antenna is developed based on cavity model. The proposed design is fabricated and various antenna parameters are measured. The details of antenna design and results are discussed in the following sections.
II. ANTENNA DESIGN AND GEOMETRICAL CONFIGURATION
The top view and the side view of the proposed antenna configuration are shown in Fig. 1. The radiating patch is printed on lower side of a substrate of thickness h2. On the other side a conducting strip of dimension ls × ws is printed. The relative position of the strip is at a distance ‘p' from the edge of the patch. This strip is excited by center conductor of coaxial probe. The patch is suspended at a height h1 from the ground plane. An inverted U-slot of dimensions L2 × W2 with width d1 is etched within another U-slot of dimensions L1 × W1 with same width d1. This DUSPA which is printed on the lower side of the substrate of thickness h2, is energized by a conducting strip printed on the upper side of this substrate. Further two parasitic elements of dimensions Lp × Wp separated by gap D are placed at thickness h3 from the conducting strip. These parasitic patches are excited by electromagnetic coupling with DUSPA. A detail design specification is given in Table-1.
Fig. 1 Geometry of the proposed antenna (a) Top view (b) Side view.
Table 1 Design specifications of the proposed antenna:
Parameter | Value |
---|---|
Wg × Lg | 80 × 52 mm2 |
W × L | 70 × 48 mm2 |
W1 × L1 | 42 × 29 mm2 |
W2 × L2 | 30 × 23 mm2 |
c × d | 10 × 8 mm2 |
Air gap (h1) | 3.5 mm |
Height of dielectric substrate (h2) | 1.55 mm |
Height of dielectric substrate (h3) | 1.50 mm |
Substrate used | RT/duriod 5880 (εr = 2.2) |
Conducting strip (ws × ls) | 3.0 × 10.5 mm2 |
Wp × Lp | 31.2 × 25 mm2 |
d1 | 1.0 mm |
W3 | 5.0 mm |
W4 | 28.0 mm |
W5 | 14.0 mm |
D | 5.6 mm |
H | 6.85 mm |
l3 | 7.0 mm |
p | 5.0 mm |
The proposed antenna is fabricated on Rogers RT duriod substrate (dielectric constant 2.2) with ground plane dimensions (Wg × Lg) 80 × 52 mm2 (Fig. 2).
U Slot Patch Antenna Calculator Download
Fig. 2 Photograph of proposed fabricated antenna.
III. THEORETICAL INVESTIGATION
(a) Analysis of modified feeding
Modified L-probe feeding can be analyzed into two parts i.e. vertical probe and horizontal strip.
Vertical Probe

Vertical probe can be analyzed as series combination of resistance Rv and inductance Lv, and can be given as [19].
where μ = permeability of the probe conductor, f = frequency in GHz, dp = diameter of probe
and
Horizontal Strip
A series combination of distributive resistance Rs and inductive Ls is developed due to horizontal conducting strip and can be given as [20].
here, ws = width of strip, ts = thickness of strip, ρ0 = ratio of specific resistance of strip and copper.
The distributive capacitance Csp between horizontal strip and the radiating patch can be given as
Since the open ends of the horizontal strip above the radiating patch will have fringing field, so the effective length of the strip is increased. The increment of length will cause some extra capacitance which is fringing capacitance and it can be calculated as
here,
in which εes is effective dielectric constant for the material under conducting strip [19]. The fringing capacitance Cfp between open end of the strip and the radiating patch is calculated by putting the substrate height h = h2 and the fringing capacitance Cfg can be given by putting h = (h1 + h2). The fringing capacitance Cpf1 between the parasitic elements and the strip is calculated by putting h = h3. The entire feeding acts as a series L-C resonant element and connected in series with the radiating patch. The impedance of this modified L-probe can be calculated using Fig. 3 as
where,
(b) Analysis for DUSPA
The value of capacitance C1, inductance L1 and resistance R1 for a rectangular patch can be given as [20].

where, L = length of the patch, W = width of the patch, f1 = resonance frequency, ls = feed point location, ω = 2πf1 and Qr is the quality factor of the resonator.
where, c = velocity of light, f1 = design frequency, εe = effective permittivity of the medium
in which, εrs can be calculated as
where, n is the number of stacked layers and εr = relative permittivity of the substrate material.
A slot in the radiating structure can be analyzed using the duality relationship between the dipole and slot [21]. The radiation resistance of an inclined slot in the patch is given by
The input reactance of the inclined slot is given as [22]
here, α is the inclination angle of slot with respect to x-axis, Si and Ci are the sine and cosine integrals, d1 = thickness of the slot, L1 = length of the slot. Impedance for this inclined slot is given by [22]
here
Now, U-slot in a patch is analyzed by assuming two slots along the y-axis as the vertical slot of length ‘L1' at angle α = 00 and a slot along x-axis as the horizontal slot of dimension ‘W1' at angle α = 900.
The input impedance of the vertical slot can be calculated by using equations (9), (10) and (11) as:
here, ZV1 is calculated by putting α = 00. Similarly the impedance of the horizontal slot can be calculated as:
here, ZH1 is calculated by putting α = 900. Thus equivalent circuit for U-slot in the patch is given by Fig. 4.
2 Meter Slot Antenna
Fig. 4 Equivalent circuit of U-slot in the patch.
Thus, the equivalent circuit for modified L-probe fed patch can be given as shown in Fig. 5.
The input impedance of DUSPA is calculated by using Fig. 5 as:
in which, ZU1 and ZU2 are the impedances of two U-slots in the patch and can be calculated using Fig. 4 and ZP is the impedance of the rectangular patch and can be calculated as:
(c) Analysis of the parasitic elements
The parasitic elements are excited through the electromagnetic coupling with DUSPA. Each parasitic element is considered equivalent to parallel combination of resistance Rp, Lp and Cp. These two parasitic elements are coupled with each other by the gap coupling and the equivalent circuit is given in Fig. 6. The equivalent circuit of the gap can be given as a π-circuit, consisting of the gap coupling capacitance Cg and the plate capacitances CP1. Now two radiating structures (DUSPA and parasitic elements) are coupled through the electromagnetic coupling.
Fig. 6 Equivalent circuit for the gap coupled parasitic elements.
The accurate equations for the coupling capacitance Cg and the plate capacitances CP1 of the microstrip gap can be calculated from the hybrid mode analysis [23], [24]. Now using the equivalent circuit as shown in Fig. 7, the total input impedance of DUSPA with parasitic elements can be calculated as:
and
in which,
here, LM, CM are the mutual coupling inductance and capacitance between two radiators and ZP1 is the impedance of parasitic elements.
Fig. 7 Equivalent circuit of the modified L-probe fed DUSPA with parasitic elements.
Using equation (16), we can calculate the reflection coefficient, VSWR and return loss of the proposed antennas.
where, Z0= characteristic impedance of the coaxial feed (50 Ω)
and Poker medicine.
IV. Result and discussion
Fig. 8 shows the simulated return loss obtained from CST Microwave studio for different values of dual U-slot width (d1). From the graph it is observed that entire operating band shifts towards higher side for increasing value of d1, however, antenna bandwidth decreases with increasing value of d1. The bandwidth of the antenna is calculated for return loss < −10 dB and found maximum (16.67%, 2.66 − 3.14 GHz) at d1 = 1.0 mm and below d1 = 1.0 mm the antenna exhibits dual band nature.
Fig. 8 Variation of return loss with frequency for different slot thickness (d1).
A comprehensive analysis has been done to study the bandwidth variation of DUSPA for different combination of dual U-slot length L1, L2 and slot width d1. From Fig. 9, it is observed that the bandwidth of the antenna increases with increasing value of slot length L1. The maximum bandwidth (16.67%) is obtained at d1 = 1.0 mm and L1 = 30.0 mm. Fig. 10 reveals the similar variation of bandwidth as observed in Fig. 9. The highest bandwidth (16.93%, 2.65 to 3.14 GHz) is seen when d1 = 1.0 mm, L1 = 30.0 mm and L2 = 24.0 mm.
Fig. 9 Bandwidth variation of DUSPA for different (a) outer slot length L1 (b) inner slot length L2.
Fig. 10 Bandwidth variation of DUSPA for combination of L1 and L2.
In the process of improving the antenna bandwidth, two parasitic elements are placed at a distance 3.05 mm from DUSPA. Fig. 11 shows the variation of return loss with frequency for different values of parasitic patch length (Lp). It is observed that the bandwidth is almost invariant with Lp. The optimum bandwidth achieved is 25.26 % varying from 2.88 to 3.71 GHz at Lp = 26.0 mm. However, when Lp = 27.0 mm, the antenna exhibits dual nature.
Fig. 11 Variation of return loss with frequency for different values of Lp.
From Fig. 12, it is observed that bandwidth decreases as gap D increases. The maximum bandwidth obtained is 24.59% (from 2.92 to 3.74 GHz) at D = 5.0 mm. At the higher value of D, the gap coupling effect between two parasitic elements is ceases to exist and the antenna starts behaving like DUSPA. Below D < 5.0 mm the increment in the bandwidth is almost constant.
Fig. 12 Variation of return loss with frequency for different value of gap ‘D'.
From Fig. 13, it is observed that the bandwidth of the antenna increases with decreasing the value of Wp. From this graph it is observed that the highest operating bandwidth of 25.89% (from 2.90 to 3.77 GHz) is achieved for the optimized value of Wp = 31.2 mm. Further, below 31.2 mm the antenna shows dual nature.
Fig. 13 Variation of return loss with frequency for different values of Wp.
The simulated return loss is compared with the theoretical and the measured results of DUSPA and DUSPA with parasitic elements respectively (Fig. 14). From the graph it is clear that simulated and theoretical results of both the antennas are agreeing quiet well with the measured one. Fig. 15 depicts the simulated and measured gain for both the antennas. The simulated peak gain for DUSPA is 8.23 dBi at 2.8 GHz while for DUSPA with parasitic elements peak gain is 8.46 dBi at 3.0 GHz. For DUSPA, the maximum gain variation is 0.63 dBi for the entire band of operation (2.75-3.10 GHz) while for DUSPA with parasitic elements it is 0.96 dBi for the entire band of operation (2.82-3.75 GHz).
Fig. 14 Measured return loss for DUSPA and DUSPA with parasitic elements.
Fig. 15 Measured gain for DUSPA and DUSPA with parasitic elements.
Radiation efficiency is calculated for both the antennas and found quite acceptable (above 97.0%) for entire operating frequency band (Fig. 16). The radiation patterns of the proposed antennas are measured using anechoic chamber. Fig. 17 shows the measured and simulated radiation patterns for DUSPA at 2.80 GHz and 3.09 GHz. The cross polarization level is quite low at φ = 900 than that at φ = 00. A good agreement between the measured and simulated results is observed. Radiation patterns for DUSPA with parasitic elements are plotted at 3.09 GHz, 3.45 GHz and 3.63 GHz (Fig. 18). The cross polarization level at φ = 900 is again quite low than that at φ = 00 for all three resonant frequencies. This is primarily because of the feed location which is along y-axis. Also, the inherent asymmetry property of probe feed which generates higher order modes and hence increases the cross-polarization level. In the measured results, some ripples are observed below ground plane due to reflection of radiation by the conducting strip. The simulated radiation pattern is compared with measured results however, some mismatch is observed in radiation pattern due to fabrication inaccuracy and numerical methods used in simulator.
Fig. 16 Simulated radiation efficiency for DUSPA and DUSPA with parasitic elements.
Fig. 17 Radiation pattern of DUSPA at (a) 2.80 GHz, and (b) 3.09 GHz.
Fig. 18 Radiation pattern of DUSPA with parasitic elements at (a) 3.09 GHz, (b) 3.45 GHz, and (c) 3.63 GHz.
It is observed that all simulated co-polar graphs are showing almost semi circular back lobe bellow the ground plane. It may be due to the size and thickness limitation of the ground plane taken in our design for the simulation. Both the antennas exhibit linearly polarized, broadside and symmetrical radiation patterns for entire operating frequency band.
V. CONCLUSION
A dual U-slot loaded patch with a modified L-strip feeding technique is presented. The equivalent circuit model is successfully implemented for the calculation of various antenna parameters. The modification in the feeding technique makes the antenna fabrication convenient as compared to proximity coupled L-strip feeding. From this investigation it is inferred that L-probe fed dual U-slot loaded patch increases the bandwidth up to 16.93%. Moreover, when DUSPA is stacked with two parasitic elements, the gap (D) between the two parasitic elements and width Wp are the key parameters to increase the bandwidth. In this stacked configuration the antenna bandwidth increases up to 25.89% with maximum gain of 8.46 dBi. The dimension of conducting strip can be optimized to further improve the antenna characteristics. Antenna bandwidth can also be controlled with inner and outer U-slot dimensions. This antenna is operating in S-band which can be used in weather radar, surface ship radar and other communication satellites.
REFERENCES
[1] K.L. Wong, Compact and Broadband Microstrip Antennas, New York, Wiley, 2002. [ Links ]
[2] G. Kumar and K. P. Ray, Broadband Microstrip Antennas, Artech House, Norwood, MA, 2003. [ Links ]
[3] B. L. Ooi, 'A double-π stub proximity feed U-slot patch antenna,' IEEE Trans. Antennas Propag., 52, (2004), 2491-2496. [ Links ]
[4] Y. Sung, 'Printed Wide-Slot Antenna With a Parasitic Center Patch,' IEEE Trans. Antennas Propag., 60 (2012), 1712-1716. [ Links ]
[5] Y. Chen, S. Yang, and Z. Nie, 'Bandwidth enhancement method for low profile E-shaped microstrip patch antennas,' IEEE Trans. Antennas Propag., 58, (2010), 2442-2447. [ Links ]
[6] Y. Chen, and C. F. Wang, 'Characteristic-mode-based improvement of circularly polarized U-slot and E-shaped patch antennas,' IEEE Antennas Wirel. Propag. Lett., 11 (2012), 1474-1477. [ Links ]
[7] S. Bhardwaj, and R. Y. Samii, 'A comparative study of C-shaped, E-shaped, and U-slotted patch antennas,' Microw. Opt. Technol. Lett., 54 (2012), 1746-1757. [ Links ]
[8] K. F. Lee, S. L. S. Yang, A. A. Kishk, and K. M. Luk, 'The versatile U-slot patch,' IEEE Antennas and Propag. Magaz., 52 (2010), 71-88. [ Links ]
[9] Z. Wang, S. Fang, and S. Fu, 'Wideband dual-layer patch antenna fed by a modified L-strip,' Journal of Microw. Opt. and Electro. Applic., 9, (2010), 89-100. [ Links ]
[10] A. A. Deshmukh, and K. P. Ray, 'Broadband proximity-fed modified rectangular microstrip antenna,' IEEE Antenna Propag. Mag., 53 (2011), 1-5. [ Links ]
[11] L. Xu, L. Li, and W. Zhang, 'Study and design of broadband bow-tie slot antenna fed with asymmetric CPW,' IEEE Trans. Antenna Propag., 63 (2015), 760-765. [ Links ]
[12] J. A. Ansari, P. Singh, and N. P. Yadav, 'Analysis of wideband multilayer patch antenna with two parasitic elements,' Microw. Opt. Technol. Lett., 51 (2009), 1397-1401. [ Links ]
[13] N. Safa Nafea, Alyani Ismail, and Raja S. A. Raja Abdullah, 'Low side lobe level multilayer antenna for wireless applications,' Progress In Electromagnetics Research Letters, 58 (2016), 105–111. [ Links ]
[14] W.-L. Chen, G.-M. Wang, and C.X. Zhang, 'Bandwidth enhancement of a microstrip-line-fed printed wide-slot antenna with a fractal-shaped slot,' IEEE Trans. Antennas Propag., 57 (2009), 2176–2179. [ Links ]
[15] J.-Y. Jan, and L.-C. Tseng, 'Small planar monopole antenna with a shorted parasitic inverted-L wire for wireless communications in the 2.4, 5.2, and 5.8-GHz bands,' IEEE Trans. Antennas Propag., 52 (2004), 1903–1905. [ Links ]
[16] J. H. Lee, and J. G. Yook, 'Improvement of radiation performance of mobile phone antenna using parasitic element,' IEEE Trans. Cons. Electron., 56 (2010), 2411–2415. [ Links ]
Square Patch Antenna Calculator
[17] Tsien-Ming Au, Kin-Fai Tong, and Kwai-Man Luk, 'Theoretical and experimental studies of a microstrip antenna with two parasitic patches,' Int J RF and Microwave CAE, 8 (1998), 49–55. [ Links ]
Patch Antenna Design Calculator
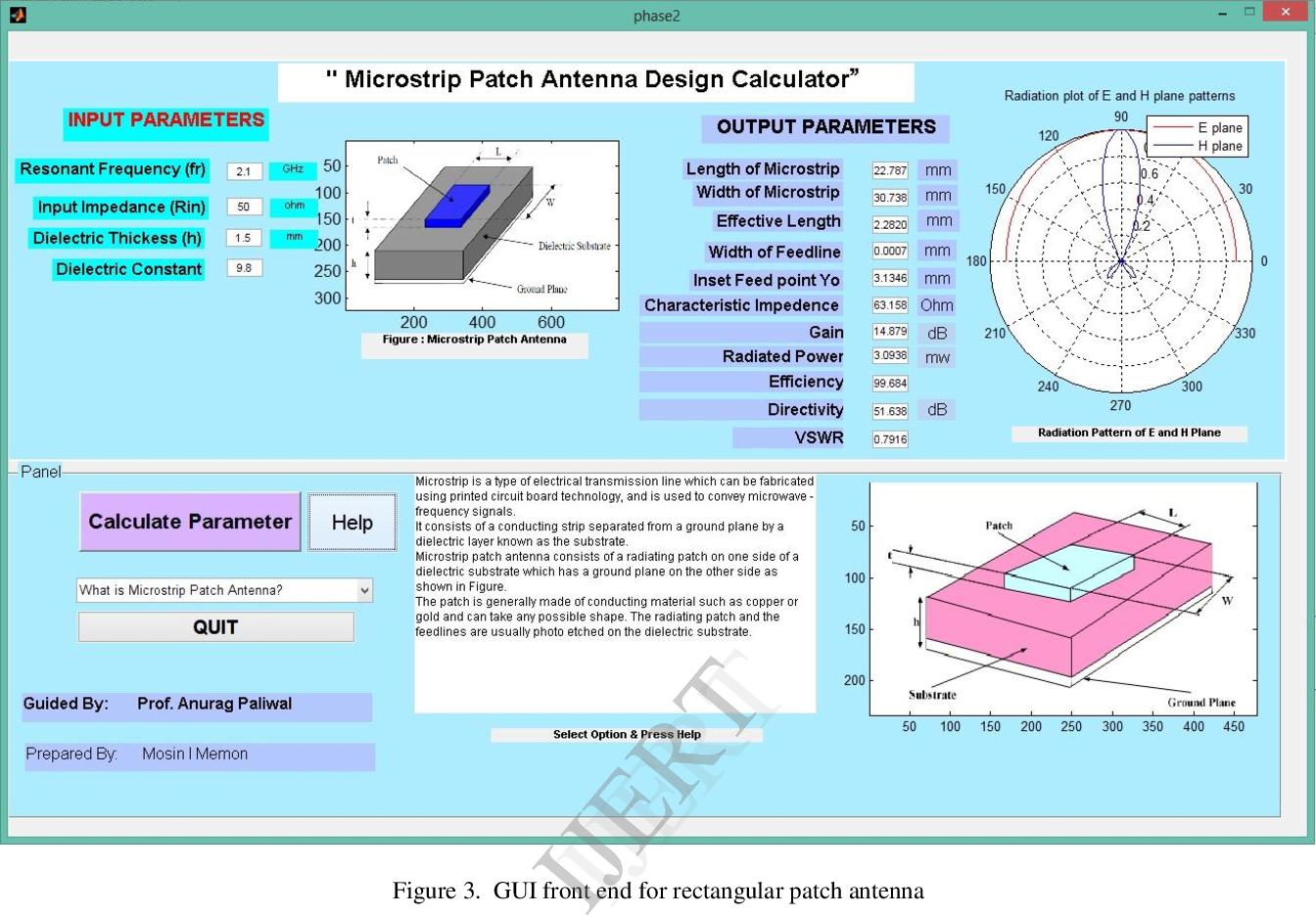
Q: How are antennas designed?
A: Decades ago, an antenna design started with an idea for the configuration and some basic analysis based on Maxwell's equations for the sizing of antenna elements, followed by building and testing. In recent years, the design mode has shifted radically to the designer establishing a configuration and then modeling it in detail using an 'EM field solver' application. These advanced applications take into account the real-world factors such as fringing and parasitics, which affect antenna dimensions, configuration, and performance, and which were nearly impossible to assess in advance using 'rules of thumb' and experience alone.
However, these advanced analysis and modeling applications cannot suggest radically new configurations; in most cases, that is done by leveraging and expanding on existing approaches or the intuition or experience of the designer. These hunches can then be verified and fine-tuned by the EM field-solver software and extended to 'what if?' modes and sensitivity analysis.
Q: What is a microstrip antenna?
A: A microstrip antenna, also called a patch antenna or printed antenna, is an antenna which is primarily a two-dimensional flat structure (Figure 6. In its simplest form, it uses a conducting 'patch' one-half wavelength long, so that the metal surface acts as a resonator similarly to the half-wave dipole antennas.
Q: Is that it, or is there more to the story?
A: Of course there is more! It is often built by simply mounting or fabricating a suitably dimensioned metal sheet or surface on an insulating dielectric substrate, such as a printed circuit board. The opposite side of the PC board substrate is also cladded and so forms a ground plane, or another conductive surface is added ion the other side of the dielectric. As a result, it is easy to design and inexpensive to manufacture. They can be built as standalone, discrete devices as a 'sandwich' of conductive material, dielectric, and conductive material as the ground plane.
Microstrip antennas are useful about 500 MHz to as high as 100 GHz; at the lower frequencies, they can be fairly large due to their half-wavelength dimension. As with many simple antennas, the basic EM theory and Maxwell's equations needed to model them start simple, but fringing, physical reality, tolerances, and parasitics play a large role in their actual performance.
Part 2 of this FAQ will explore the microstrip antenna in more detail, including its primary attributes, advantages and disadvantages, and variations.
EE World Related Content
Take a class in SDR and antenna design
The difference between active and passive antenna systems
Hidden pitfalls of IoT antenna design
New Antenna Concept Developed for Cars
What are RF waveguides? Part 2: implementation and components
What are RF waveguides? Part 1: context and principles
5 things you need to know about 5G filters
Passive microwave components, Part 2: couplers and splitters
Passive microwave components, Part 1: isolators and circulators
How do RFID tags and reader antennas work?
References and Resources
Basics and tutorials
- Maxtena, 'Microstrip Patch Antennas'
- IOP Conference Series: Materials Science and Engineering, 'Design, simulation and analysis: a microstrip antenna using PU-EFB substrate'
- Pasternack, 'Microstrip Patch Antenna Calculator'
- EU Radar Tutorial, 'Patch Antenna or Microstrip Antenna'
- Ranga Rodrigo, Arab Academy for Science, Technology, and Maritime Transport, 'Fundamental Parameters of Antennas'
- Pongsak University, Thailand, 'Chapter 2 : Fundamental parameters of antennas
- Tutorials Point, 'Antenna Theory – Micro Strip'
- Universal Engineering College, University of Calicut, 'Radiation and Propagation: Fundamentals of Antenna'
- ResearchGate, 'An Overview Of Microstrip Antenna'
- ResearchGate, 'Design of rectangular microstrip patch antenna'
- ResearchGate, 'Methods to Design Microstrip Antennas for Modern Applications'
- Science Direct, 'Microstrip Antenna'
- Science Direct, 'Stripline'
- Antenna-Theory.com, 'Microstrip (Patch) Antennas'
- Antenna-Theory.com,'PIFA – The Planar Inverted-F Antenna'
Advanced microstrip antenna implementations
- Elsevier, Science Direct, 'A Novel Compact Dual Frequency Microstrip Antenna'
- Elsevier, Journal of Applied Research and Technology, 'Design of triple-layer double U-slot patch antenna for wireless applications'
- ResearchGate, 'Methods to Design Microstrip Antennas for Modern Applications'
You may also like:
Services on Demand
- Cited by SciELO
Related links
- Similars in SciELO
On-line version ISSN 2179-1074
http://dx.doi.org/10.1590/2179-10742017v16i3867
Articles
Dual U-Slot Loaded Patch Antenna with a Modified L-Probe Feeding
1Department of Electronics and Communication Engineering, Raffles University, Neemrana, Rajasthan, INDIA-301705, Email: srakeshnath@gmail.com
2Department of Physics, Galgotias University, Greater Noida, Uttar Pradesh, INDIA-201308, Email: prabhakarsingh3@gmail.com
3School of Computational and Integrative Sciences, Jawaharlal Nehru University, New Delhi, INDIA-110067, Email: bkkanaujia@yahoo.co.in
In this paper, a modified L-strip fed patch antenna is theoretically analyzed for wideband applications. Dual U-shaped slots are incorporated in the radiating patch and a maximum bandwidth of 16.93% (2.65 GHz to 3.14 GHz) is achieved. Further, when two parasitic elements are used, antenna bandwidth improves up to 25.89% (2.90 GHz to 3.77 GHz). The maximum gain of dual U-slotted patch and with parasitic elements is 8.23 dBi and 8.46 dBi respectively. Antenna parameters are calculated by CST Microwave studio and equivalent circuit model theory is presented. The proposed antenna is fabricated and the measured results compare well with the theoretical as well as simulated results.
Index Terms Dual U-slot patch antenna; modified L-probe; multilayer patch antenna; gap coupled patch antenna; parasitic elements
I. INTRODUCTION
With the rapid development and attractive solution for various wireless communication systems and demands of their applications, compact and wideband antenna designs have been given immense priority [1], [2]. In this process, various methods were used to improve the bandwidth such as by loading the slots of different size and shape, etching notches and introducing discontinuities in the radiating patch as well as in the ground plane [3], [4]. There are several structures reported to improve the antenna characteristics such as E-shaped [5], [6], C-shaped, U-slot loaded and modified L-strip [7-9]. Different feeding methods also increase the antenna bandwidth such as proximity feed patch, asymmetric CPW fed patch antennas [10], [11]. Substrate with low dielectric constants, multilayer structures and use of air gaps between the dielectric layers increases the impedance bandwidth and gain of the microstrip antennas [12-14]. Besides the fed patch, some parasitic inverted-L wire improves the radiation performance of patch antennas [15], [16]. Apart from that some other types of parasitic element design such as slot type, shorted strip type etc [17], [18] can improve the antenna bandwidth and gain.
The present paper reports a radiating structure to study the antenna bandwidth, gain, efficiency and the radiation pattern. Dual U-slot is incorporated in the radiating patch such that one U-slot is lying within another U-slot forming dual U-slot loaded patch antenna (DUSPA). Further, to increase the bandwidth, two parasitic elements are used above this DUSPA. Both designs are fed by modified L-probe. All the calculations are made by using CST Microwave studio. Also, a theoretical analysis for the proposed antenna is developed based on cavity model. The proposed design is fabricated and various antenna parameters are measured. The details of antenna design and results are discussed in the following sections.
II. ANTENNA DESIGN AND GEOMETRICAL CONFIGURATION
The top view and the side view of the proposed antenna configuration are shown in Fig. 1. The radiating patch is printed on lower side of a substrate of thickness h2. On the other side a conducting strip of dimension ls × ws is printed. The relative position of the strip is at a distance ‘p' from the edge of the patch. This strip is excited by center conductor of coaxial probe. The patch is suspended at a height h1 from the ground plane. An inverted U-slot of dimensions L2 × W2 with width d1 is etched within another U-slot of dimensions L1 × W1 with same width d1. This DUSPA which is printed on the lower side of the substrate of thickness h2, is energized by a conducting strip printed on the upper side of this substrate. Further two parasitic elements of dimensions Lp × Wp separated by gap D are placed at thickness h3 from the conducting strip. These parasitic patches are excited by electromagnetic coupling with DUSPA. A detail design specification is given in Table-1.
Fig. 1 Geometry of the proposed antenna (a) Top view (b) Side view.
Table 1 Design specifications of the proposed antenna:
Parameter | Value |
---|---|
Wg × Lg | 80 × 52 mm2 |
W × L | 70 × 48 mm2 |
W1 × L1 | 42 × 29 mm2 |
W2 × L2 | 30 × 23 mm2 |
c × d | 10 × 8 mm2 |
Air gap (h1) | 3.5 mm |
Height of dielectric substrate (h2) | 1.55 mm |
Height of dielectric substrate (h3) | 1.50 mm |
Substrate used | RT/duriod 5880 (εr = 2.2) |
Conducting strip (ws × ls) | 3.0 × 10.5 mm2 |
Wp × Lp | 31.2 × 25 mm2 |
d1 | 1.0 mm |
W3 | 5.0 mm |
W4 | 28.0 mm |
W5 | 14.0 mm |
D | 5.6 mm |
H | 6.85 mm |
l3 | 7.0 mm |
p | 5.0 mm |
The proposed antenna is fabricated on Rogers RT duriod substrate (dielectric constant 2.2) with ground plane dimensions (Wg × Lg) 80 × 52 mm2 (Fig. 2).
U Slot Patch Antenna Calculator Download
Fig. 2 Photograph of proposed fabricated antenna.
III. THEORETICAL INVESTIGATION
(a) Analysis of modified feeding
Modified L-probe feeding can be analyzed into two parts i.e. vertical probe and horizontal strip.
Vertical Probe
Vertical probe can be analyzed as series combination of resistance Rv and inductance Lv, and can be given as [19].
where μ = permeability of the probe conductor, f = frequency in GHz, dp = diameter of probe
and
Horizontal Strip
A series combination of distributive resistance Rs and inductive Ls is developed due to horizontal conducting strip and can be given as [20].
here, ws = width of strip, ts = thickness of strip, ρ0 = ratio of specific resistance of strip and copper.
The distributive capacitance Csp between horizontal strip and the radiating patch can be given as
Since the open ends of the horizontal strip above the radiating patch will have fringing field, so the effective length of the strip is increased. The increment of length will cause some extra capacitance which is fringing capacitance and it can be calculated as
here,
in which εes is effective dielectric constant for the material under conducting strip [19]. The fringing capacitance Cfp between open end of the strip and the radiating patch is calculated by putting the substrate height h = h2 and the fringing capacitance Cfg can be given by putting h = (h1 + h2). The fringing capacitance Cpf1 between the parasitic elements and the strip is calculated by putting h = h3. The entire feeding acts as a series L-C resonant element and connected in series with the radiating patch. The impedance of this modified L-probe can be calculated using Fig. 3 as
where,
(b) Analysis for DUSPA
The value of capacitance C1, inductance L1 and resistance R1 for a rectangular patch can be given as [20].
where, L = length of the patch, W = width of the patch, f1 = resonance frequency, ls = feed point location, ω = 2πf1 and Qr is the quality factor of the resonator.
where, c = velocity of light, f1 = design frequency, εe = effective permittivity of the medium
in which, εrs can be calculated as
where, n is the number of stacked layers and εr = relative permittivity of the substrate material.
A slot in the radiating structure can be analyzed using the duality relationship between the dipole and slot [21]. The radiation resistance of an inclined slot in the patch is given by
The input reactance of the inclined slot is given as [22]
here, α is the inclination angle of slot with respect to x-axis, Si and Ci are the sine and cosine integrals, d1 = thickness of the slot, L1 = length of the slot. Impedance for this inclined slot is given by [22]
here
Now, U-slot in a patch is analyzed by assuming two slots along the y-axis as the vertical slot of length ‘L1' at angle α = 00 and a slot along x-axis as the horizontal slot of dimension ‘W1' at angle α = 900.
The input impedance of the vertical slot can be calculated by using equations (9), (10) and (11) as:
here, ZV1 is calculated by putting α = 00. Similarly the impedance of the horizontal slot can be calculated as:
here, ZH1 is calculated by putting α = 900. Thus equivalent circuit for U-slot in the patch is given by Fig. 4.
2 Meter Slot Antenna
Fig. 4 Equivalent circuit of U-slot in the patch.
Thus, the equivalent circuit for modified L-probe fed patch can be given as shown in Fig. 5.
The input impedance of DUSPA is calculated by using Fig. 5 as:
in which, ZU1 and ZU2 are the impedances of two U-slots in the patch and can be calculated using Fig. 4 and ZP is the impedance of the rectangular patch and can be calculated as:
(c) Analysis of the parasitic elements
The parasitic elements are excited through the electromagnetic coupling with DUSPA. Each parasitic element is considered equivalent to parallel combination of resistance Rp, Lp and Cp. These two parasitic elements are coupled with each other by the gap coupling and the equivalent circuit is given in Fig. 6. The equivalent circuit of the gap can be given as a π-circuit, consisting of the gap coupling capacitance Cg and the plate capacitances CP1. Now two radiating structures (DUSPA and parasitic elements) are coupled through the electromagnetic coupling.
Fig. 6 Equivalent circuit for the gap coupled parasitic elements.
The accurate equations for the coupling capacitance Cg and the plate capacitances CP1 of the microstrip gap can be calculated from the hybrid mode analysis [23], [24]. Now using the equivalent circuit as shown in Fig. 7, the total input impedance of DUSPA with parasitic elements can be calculated as:
and
in which,
here, LM, CM are the mutual coupling inductance and capacitance between two radiators and ZP1 is the impedance of parasitic elements.
Fig. 7 Equivalent circuit of the modified L-probe fed DUSPA with parasitic elements.
Using equation (16), we can calculate the reflection coefficient, VSWR and return loss of the proposed antennas.
where, Z0= characteristic impedance of the coaxial feed (50 Ω)
and Poker medicine.
IV. Result and discussion
Fig. 8 shows the simulated return loss obtained from CST Microwave studio for different values of dual U-slot width (d1). From the graph it is observed that entire operating band shifts towards higher side for increasing value of d1, however, antenna bandwidth decreases with increasing value of d1. The bandwidth of the antenna is calculated for return loss < −10 dB and found maximum (16.67%, 2.66 − 3.14 GHz) at d1 = 1.0 mm and below d1 = 1.0 mm the antenna exhibits dual band nature.
Fig. 8 Variation of return loss with frequency for different slot thickness (d1).
A comprehensive analysis has been done to study the bandwidth variation of DUSPA for different combination of dual U-slot length L1, L2 and slot width d1. From Fig. 9, it is observed that the bandwidth of the antenna increases with increasing value of slot length L1. The maximum bandwidth (16.67%) is obtained at d1 = 1.0 mm and L1 = 30.0 mm. Fig. 10 reveals the similar variation of bandwidth as observed in Fig. 9. The highest bandwidth (16.93%, 2.65 to 3.14 GHz) is seen when d1 = 1.0 mm, L1 = 30.0 mm and L2 = 24.0 mm.
Fig. 9 Bandwidth variation of DUSPA for different (a) outer slot length L1 (b) inner slot length L2.
Fig. 10 Bandwidth variation of DUSPA for combination of L1 and L2.
In the process of improving the antenna bandwidth, two parasitic elements are placed at a distance 3.05 mm from DUSPA. Fig. 11 shows the variation of return loss with frequency for different values of parasitic patch length (Lp). It is observed that the bandwidth is almost invariant with Lp. The optimum bandwidth achieved is 25.26 % varying from 2.88 to 3.71 GHz at Lp = 26.0 mm. However, when Lp = 27.0 mm, the antenna exhibits dual nature.
Fig. 11 Variation of return loss with frequency for different values of Lp.
From Fig. 12, it is observed that bandwidth decreases as gap D increases. The maximum bandwidth obtained is 24.59% (from 2.92 to 3.74 GHz) at D = 5.0 mm. At the higher value of D, the gap coupling effect between two parasitic elements is ceases to exist and the antenna starts behaving like DUSPA. Below D < 5.0 mm the increment in the bandwidth is almost constant.
Fig. 12 Variation of return loss with frequency for different value of gap ‘D'.
From Fig. 13, it is observed that the bandwidth of the antenna increases with decreasing the value of Wp. From this graph it is observed that the highest operating bandwidth of 25.89% (from 2.90 to 3.77 GHz) is achieved for the optimized value of Wp = 31.2 mm. Further, below 31.2 mm the antenna shows dual nature.
Fig. 13 Variation of return loss with frequency for different values of Wp.
The simulated return loss is compared with the theoretical and the measured results of DUSPA and DUSPA with parasitic elements respectively (Fig. 14). From the graph it is clear that simulated and theoretical results of both the antennas are agreeing quiet well with the measured one. Fig. 15 depicts the simulated and measured gain for both the antennas. The simulated peak gain for DUSPA is 8.23 dBi at 2.8 GHz while for DUSPA with parasitic elements peak gain is 8.46 dBi at 3.0 GHz. For DUSPA, the maximum gain variation is 0.63 dBi for the entire band of operation (2.75-3.10 GHz) while for DUSPA with parasitic elements it is 0.96 dBi for the entire band of operation (2.82-3.75 GHz).
Fig. 14 Measured return loss for DUSPA and DUSPA with parasitic elements.
Fig. 15 Measured gain for DUSPA and DUSPA with parasitic elements.
Radiation efficiency is calculated for both the antennas and found quite acceptable (above 97.0%) for entire operating frequency band (Fig. 16). The radiation patterns of the proposed antennas are measured using anechoic chamber. Fig. 17 shows the measured and simulated radiation patterns for DUSPA at 2.80 GHz and 3.09 GHz. The cross polarization level is quite low at φ = 900 than that at φ = 00. A good agreement between the measured and simulated results is observed. Radiation patterns for DUSPA with parasitic elements are plotted at 3.09 GHz, 3.45 GHz and 3.63 GHz (Fig. 18). The cross polarization level at φ = 900 is again quite low than that at φ = 00 for all three resonant frequencies. This is primarily because of the feed location which is along y-axis. Also, the inherent asymmetry property of probe feed which generates higher order modes and hence increases the cross-polarization level. In the measured results, some ripples are observed below ground plane due to reflection of radiation by the conducting strip. The simulated radiation pattern is compared with measured results however, some mismatch is observed in radiation pattern due to fabrication inaccuracy and numerical methods used in simulator.
Fig. 16 Simulated radiation efficiency for DUSPA and DUSPA with parasitic elements.
Fig. 17 Radiation pattern of DUSPA at (a) 2.80 GHz, and (b) 3.09 GHz.
Fig. 18 Radiation pattern of DUSPA with parasitic elements at (a) 3.09 GHz, (b) 3.45 GHz, and (c) 3.63 GHz.
It is observed that all simulated co-polar graphs are showing almost semi circular back lobe bellow the ground plane. It may be due to the size and thickness limitation of the ground plane taken in our design for the simulation. Both the antennas exhibit linearly polarized, broadside and symmetrical radiation patterns for entire operating frequency band.
V. CONCLUSION
A dual U-slot loaded patch with a modified L-strip feeding technique is presented. The equivalent circuit model is successfully implemented for the calculation of various antenna parameters. The modification in the feeding technique makes the antenna fabrication convenient as compared to proximity coupled L-strip feeding. From this investigation it is inferred that L-probe fed dual U-slot loaded patch increases the bandwidth up to 16.93%. Moreover, when DUSPA is stacked with two parasitic elements, the gap (D) between the two parasitic elements and width Wp are the key parameters to increase the bandwidth. In this stacked configuration the antenna bandwidth increases up to 25.89% with maximum gain of 8.46 dBi. The dimension of conducting strip can be optimized to further improve the antenna characteristics. Antenna bandwidth can also be controlled with inner and outer U-slot dimensions. This antenna is operating in S-band which can be used in weather radar, surface ship radar and other communication satellites.
REFERENCES
[1] K.L. Wong, Compact and Broadband Microstrip Antennas, New York, Wiley, 2002. [ Links ]
[2] G. Kumar and K. P. Ray, Broadband Microstrip Antennas, Artech House, Norwood, MA, 2003. [ Links ]
[3] B. L. Ooi, 'A double-π stub proximity feed U-slot patch antenna,' IEEE Trans. Antennas Propag., 52, (2004), 2491-2496. [ Links ]
[4] Y. Sung, 'Printed Wide-Slot Antenna With a Parasitic Center Patch,' IEEE Trans. Antennas Propag., 60 (2012), 1712-1716. [ Links ]
[5] Y. Chen, S. Yang, and Z. Nie, 'Bandwidth enhancement method for low profile E-shaped microstrip patch antennas,' IEEE Trans. Antennas Propag., 58, (2010), 2442-2447. [ Links ]
[6] Y. Chen, and C. F. Wang, 'Characteristic-mode-based improvement of circularly polarized U-slot and E-shaped patch antennas,' IEEE Antennas Wirel. Propag. Lett., 11 (2012), 1474-1477. [ Links ]
[7] S. Bhardwaj, and R. Y. Samii, 'A comparative study of C-shaped, E-shaped, and U-slotted patch antennas,' Microw. Opt. Technol. Lett., 54 (2012), 1746-1757. [ Links ]
[8] K. F. Lee, S. L. S. Yang, A. A. Kishk, and K. M. Luk, 'The versatile U-slot patch,' IEEE Antennas and Propag. Magaz., 52 (2010), 71-88. [ Links ]
[9] Z. Wang, S. Fang, and S. Fu, 'Wideband dual-layer patch antenna fed by a modified L-strip,' Journal of Microw. Opt. and Electro. Applic., 9, (2010), 89-100. [ Links ]
[10] A. A. Deshmukh, and K. P. Ray, 'Broadband proximity-fed modified rectangular microstrip antenna,' IEEE Antenna Propag. Mag., 53 (2011), 1-5. [ Links ]
[11] L. Xu, L. Li, and W. Zhang, 'Study and design of broadband bow-tie slot antenna fed with asymmetric CPW,' IEEE Trans. Antenna Propag., 63 (2015), 760-765. [ Links ]
[12] J. A. Ansari, P. Singh, and N. P. Yadav, 'Analysis of wideband multilayer patch antenna with two parasitic elements,' Microw. Opt. Technol. Lett., 51 (2009), 1397-1401. [ Links ]
[13] N. Safa Nafea, Alyani Ismail, and Raja S. A. Raja Abdullah, 'Low side lobe level multilayer antenna for wireless applications,' Progress In Electromagnetics Research Letters, 58 (2016), 105–111. [ Links ]
[14] W.-L. Chen, G.-M. Wang, and C.X. Zhang, 'Bandwidth enhancement of a microstrip-line-fed printed wide-slot antenna with a fractal-shaped slot,' IEEE Trans. Antennas Propag., 57 (2009), 2176–2179. [ Links ]
[15] J.-Y. Jan, and L.-C. Tseng, 'Small planar monopole antenna with a shorted parasitic inverted-L wire for wireless communications in the 2.4, 5.2, and 5.8-GHz bands,' IEEE Trans. Antennas Propag., 52 (2004), 1903–1905. [ Links ]
[16] J. H. Lee, and J. G. Yook, 'Improvement of radiation performance of mobile phone antenna using parasitic element,' IEEE Trans. Cons. Electron., 56 (2010), 2411–2415. [ Links ]
Square Patch Antenna Calculator
[17] Tsien-Ming Au, Kin-Fai Tong, and Kwai-Man Luk, 'Theoretical and experimental studies of a microstrip antenna with two parasitic patches,' Int J RF and Microwave CAE, 8 (1998), 49–55. [ Links ]
Patch Antenna Design Calculator
[18] K. C. Lin, C. H. Lin, and Y. C. Lin, 'Simple printed multiband antenna with novel parasitic-elements design for multistandard mobile phone application,' IEEE Trans. Antenna Propag., 61 (2013), 488–491. [ Links ]
[19] I. J. Bahl and P. Bhartia, Microstrip Antenna. Dedham, Artech House, MA 1980. [ Links ]
[20] R. K. Hoffman, Handbook of microstrip integrated circuits, Artech House, Norwood, MA8, 1987. [ Links ]
[21] C. A. Balanis, Antenna Theory: Analysis and Design, 2nd ed. New York: Wiley, 1996. [ Links ]
[22] Shivnarayan and B. R. Vishvakarma, 'Analysis of inclined slot loaded patch for dual-band operation,' Microw. Opt. Technol. Lett., 48 (2006), 2436–2441. [ Links ]
[23] A. Gopinath and K.C. Gupta, 'Capacitance parameter of discontinuities in microstrip lines,' IEEE Trans on Microwave Theory Tech, 26 (1978), 831–836. [ Links ]
[24] N. L. Kester, and R H. Jansen, 'The equivalent circuit of the asymmetrical series gap in microstrip and suspended substrate lines,' IEEE Trans on Microwave Theory Tech, 30 (1982), 1273-1279. [ Links ]
Received: December 26, 2016; Revised: December 29, 2016; Accepted: May 26, 2017
This is an Open Access article distributed under the terms of the Creative Commons Attribution License, which permits unrestricted use, distribution, and reproduction in any medium, provided the original work is properly cited.